Introduction
Cardiovascular diseases
Coronary artery disease (CAD) is one of the most important causes of morbidity and mortality in developed countries [1]. CAD is the main reason for mortality in American men and women [2]. Although the number of deaths due to CAD has reduced in recent years, it still accounts for one-third of deaths in people over 35 [1]. Statistics in the United States also show that in the United States, almost half of middle-aged men and one-third of middle-aged women have had CAD [2]. The world health organization (WHO) reported in 2004 that cardiovascular disease and ischemic heart disease (IHD) were the leading causes of mortality worldwide. The mortality rate due to cardiovascular diseases will increase to 23.4 million cases by 2030 [3].
Atherosclerotic obstruction of coronary arteries is the preeminent cause of CAD. Atherosclerosis is a fibroproliferative and inflammatory process that responds to chronic vascular damage due to endothelial dysfunction [4].
Atherosclerosis
Atherosclerosis is associated with an inflammatory disease that can affect any vessel in the body. The occurrence of atherosclerosis in heart vessels is called CAD. In the vessel wall, endothelial cells are constantly in touch with blood flow. An essential step to starting atherosclerosis is the concentration of low-density lipoprotein (LDL) in the tunica intima [5].
Risk factors of cardiovascular disease
CAD is the prominent cause of mortality worldwide, and different genetic and environmental factors can cause them [1]. Age, gender (male), hypertension, diabetes mellitus, hyperlipidemia, smoking, positive family history of CAD, and obesity are the most significant risk factors for cardiovascular disease [3]. It seems that these risk factors have a fundamental role in the occurrence of cardiovascular disease (CVD) by creating oxidative stress [3]. In addition to these primary risk factors, genetic factors play an essential role in CAD. However, the intertwining of genetic and environmental factors, besides the relationship between genetic factors and the occurrence of CAD, is still not discovered [6].
High-density lipoprotein (HDL) and cardiovascular disease
As mentioned, CAD is related to different risk factors, and low HDL levels are among the most important [7]. For every 1% decrease in HDL, the risk of developing cardiovascular disease increases by 2-3%. Therefore, several research studies have studied the mechanisms by which HDL exerts its protective effects [8]. HDL is a complex and heterogeneous molecule, along with several circulating proteins. The well-known anti-androgenic features of HDL are related to its role as a lipid transporter in the reverse cholesterol transport pathway, a pathway in which HDL shuttles transfer excess cholesterol from peripheral tissues to the liver. In addition, HDL appears to play a role in protecting against atherosclerosis by inhibiting LDL oxidation because mane evidence shows that LDL plays an essential role in the development of atherosclerosis. The phospholipids and cholesterol in the LDL structure, located in the vessel wall's subendothelial space, undergo oxidative changes due to lipoxygenases and myeloperoxidase by-products [9]. HDL not only protects LDL against oxidation but also reduces the biological function of ox-LDL. Significantly, the oxidation of phospholipids containing arachidonic acid can produce pro-inflammatory products that trigger an immune response, including the induction of sticky molecules and the absorption of monocytes, facilitating the formation of foam-shaped cells [10]. Antioxidant and anti-atherogenic effects of HDL are related to different proteins attached [11]. Paraoxonase (PON), platelet-activating factor, acetylhydrolase, and lecithin are some associated with proteins. Acyl Transferase Cholesterol, one of the enzymes attached to cholesterol, plays an essential role in inhibiting LDL oxidation by preventing the production of lipid peroxidases [12]. PON-I is one of the proteins accompanying HDL and plays a role in HDL protective effects [13, 14].
Paraoxonase family
The PON gene family has three members, PON-I, PON-II, and PON-III. These genes are located beside each other on the long arm of chromosome-VII in humans [15]. Members of the PON family have nine exogenous with equal length, and they have about 65% similarity at the amino acid level and about 70% similarity at the nucleotide level. From an evolutionary point of view, PON-II seems to be the oldest member of the PON family, followed by PON-III and PON-I [16].
The PON-I enzyme mRNA expression is limited to the human liver, although an expression of the PON-III enzyme may happen in both liver and kidneys [17]. The PON-II enzyme gene is widely expressed in various tissues, including the heart, kidneys, liver, lungs, placenta, small intestine, spleen, and testicles. In addition, the PON-II enzyme is found in vessel wall cells, including endothelial cells, smooth muscle cells, and macrophages [18]. Studies have shown that when PON-I and PON-III enzymes are attached to HDL in the bloodstream, the PON-II enzyme is not recognizable on HDL, LDL, or cellular supernatant. Nevertheless, it seems positioned in intracellular space accompanying cell membrane segments [19].
PON-I enzyme
Recently, the PON1 enzyme has two common polymorphisms, a genetic variation resulting in several different forms or types of enzymes and proteins, both of which are single-nucleotide polymorphism (SNPs) Types. In SNP, the encoding codon of one amino acid is converted to another codon due to the replacement of one nucleotide. In L55M polymorphism, cytosine nucleotide in the CTG codon is replaced by adenine nucleotide, so a methionine amino acid is replaced by leucine at location 55 (L/M55). In Q192R polymorphism, adenine nucleotide in the CAA codon is replaced by guanine nucleotide (CGA), so arginine amino acid is replaced by glutamine at location 192 (Q/R192). The above explanations are shown in Figure 1. Both of mentioned polymorphisms are followed by some pathologic conditions. Different studies on different populations have shown that such polymorphisms can affect the levels of expression of the PON-I enzyme [20]. Besides these two polymorphisms, at least five more polymorphisms have been discovered in the PON-I enzyme promotor region [21].
Q192R polymorphism alters the ability of the PON-I enzyme to protect LDL against oxidation, while L55M polymorphism affects serum enzyme concentrations. L55M polymorphism is related to stroke, CAD, Parkinson's, and plasma levels of total cholesterol and LDL cholesterol. Besides, this polymorphism is associated with the level of mRNA expression and function of the PON-I enzyme. Q192R polymorphism can affect the hydrolysis of various substrates, including PON, diazo on, suman, and sarin.
Moreover, this polymorphism is related to CAD, stroke, hypercholesterolemia, type 2 diabetes, and Parkinson's disease [22]. An isoleucine-Valin replacement was found in location 102, with an increased risk of prostate cancer [20]. Without considering genotype, low serum function of the PON-I enzyme is accompanied by hyperlipidemia, type1 diabetes, CAD, Corticotropin-Releasing Factor (CRF), metabolic syndrome, arthrosis, thyroid gland dysfunction, and uremia [22, 23].
Q192R polymorphism has been studied in many types of research because the amino acid in location 192 can have an essential role in enzyme activity. This polymorphism can change the potential of the enzyme for protecting LDL against oxidation. Q192R polymorphism appears to alter PON enzyme activity depending on the Substrate. Some substrates, such as paraoxon, are hydrolyzed more rapidly by isoform R. In contrast, others, such as diazo, are hydrolyzed faster by isoform Q. Lately, different studies have shown that R alleles compared to Q alleles have a lesser potential for protecting LDL against oxidation due to their lower ability to hydrolyze lipid peroxides [24]. L55M polymorphism does not affect enzyme-substrate attachment but can affect serum levels and enzyme concentration. Studies have shown that individuals with MM genotype have the lowest levels of PON-I mRNA expression. As a result, the enzyme serum level in these individuals is lower than in others [25].
One of the first studies on the polymorphism of the PON enzyme was the 1997 study by Mackness et al. They purified the serum HDL of individuals with different genotypes of L55M and Q192R polymorphisms and compared their protective effects. The study showed that purified HDL from individuals with
LL genotype (PON-55 polymorphism) and RR genotype (PON-192 polymorphism) had
the lowest protective impact compared to
other genotypes. They also concluded that homozygote individuals with genotypes QQ and MM have the most effective protective activity against lipid oxidation [26]. PON-I gene and its function are shown in Figure 1.
Regulation of expression and activity of PON-I enzyme
In addition to genetic factors, it seems that environmental factors also play a role in regulating of expression and activity of the enzyme PON-I. HepG2 hepatic cell therapy by oxidized LDL, oxidized lipids, and some cytokines reduces the expression of the PON-I enzyme [27]. Smoking, frying oils used several times, and fatty acids can reduce the enzyme's activity in humans [28, 29]. However, polyphenols, vitamins C and E, and controlled alcohol consumption can increase the activity of the PON-I enzyme [30, 31].
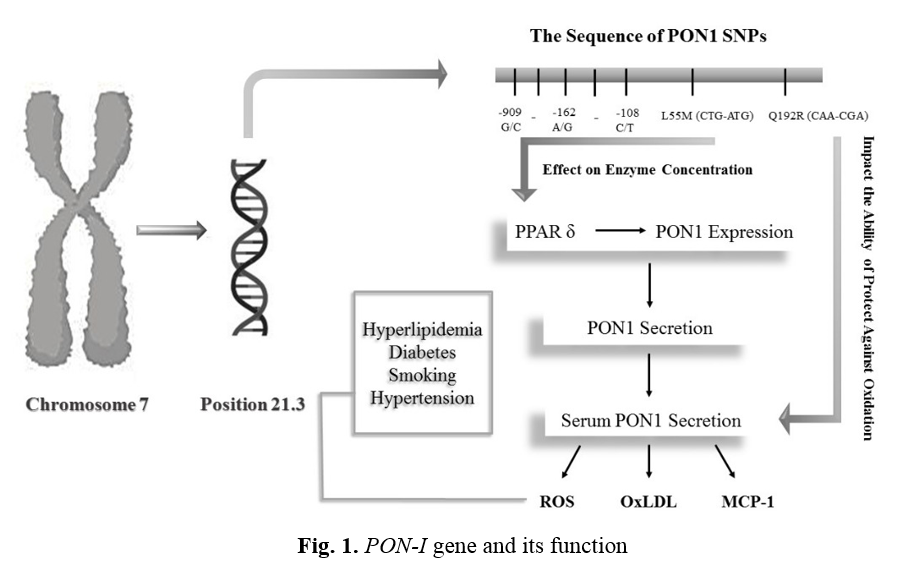
Some studies have shown that the attachment of sterol regulatory-element binding proteins (SREBP-2) regulatory protein to the proximal region of the PON-I enzyme gene promoter can increase PON-I gene expression. So one of the anti-atherogenic effects of statins can be an increase in PON-I enzyme production due to an increase in SREBP-2 gene expression [16].
Effects of fatty acids and lipid peroxidation on PON-I enzyme synthesis
The relationship between the decrease in PON-I activity and mRNA levels in the liver with atherogenic diet therapy for 8 to 14 weeks has been reported [32]. These findings suggest that changes in the enzyme PON-I serum activity indicate changes in the hepatic synthesis of this enzyme. The enzyme gene expression and other factors involved in HDL synthesis, such as cholesterol reverse transporter ATP-binding cassette transporter (ABCA1), are mediated by cell receptors depending on signals and nuclear receptors like peroxisome proliferator-activated receptors (PPARs). It appears that free radicals and lipid peroxidation products can reduce the expression of hepatic PON-I and PPARs [33].
In addition, linkages between lipid peroxidation marker levels, liver PON-I enzyme mRNA, and the expression of inflammatory genes have been reported in high-fat diets. It shows that enzyme changes are probably a part of the inflammatory response caused by lipid peroxidation [34].
A high-fat diet can cause an inflammatory response and increase the production of pro-inflammatory cytokines and reactive oxygen species by blood and hepatic cells (stage 1). As a result, lipid oxidation markers in the liver and hepatic tissue increase (stage 2). Filtration of macrophages and expansion of oxidative and inflammatory injuries are also observed (stage 3), and peroxidation products can affect the expression of the PPAR gene in hepatic cells (stage 4).
A decrease in PPAR gene expression can inhibit PON-I enzyme gene expression (stage 5) and can cause the attachment of ATP to ABCA1 receptors (stage 6). In the following stages, decreased HDL synthesis (stage 7) and reduced secretion of the PON-I enzyme (stage 8) finally happens, so the activity of this enzyme will be reduced [35].
PON-I enzyme activity and its antioxidant properties
As mentioned, the PON-I enzyme protects against LDL oxidation induced by copper or cells. Although the exact mechanism of the function of this enzyme is still unknown, studies have shown that Cysteine available in position 284 plays an essential role in the antioxidant potential of the enzyme. Aviram et al. showed that the cellular supernatant containing the enzyme PON-I, mutated in cysteine 284, could not protect LDL from oxidation [36]. Harel et al. also showed that Cysteine plays a vital role in the stability of the central structure of the protein because the mutated PON-I enzyme in this Cysteine is low expressed and unstable [37]. However, other studies have stated that the PON-I enzyme with cysteine mutation in location 284 has PON activities, so more research is needed to clarify this [38].
Discovering this fact by Mackness et al. that the enzyme PON-I in the bloodstream accompanies HDL was the starting point to studying the role of the enzyme in the metabolism of lipids and atherogenesis [39]. During the past decade, it has been discovered that PON-I can protect LDL against oxidation and inhibits the physiologic effects of oxidized LDL (oxLDL). Furthermore, this enzyme can maintain the function of HDL by inhibiting HDL oxidation [40].
Studies by Doorn et al. show that mice genetically devoid of the PON-I enzyme do not exhibit any PON plasma activity, while their heterozygous parents, compared to wild species, have 50% of PON plasma activity. In addition, when mice are fed a high fat and cholesterol diet, their atherosclerotic plaques are more significant than wild species [41]. This study and similar studies suggest that the enzyme PON-I plays a role in protecting against atherogenic factors and is a crucial component of HDL's antioxidant potential [13]. Studies by Oda et al. on mice with higher levels of PON-I enzyme have indicated that HDL taken from these mice is more resistant to peroxidation than wild species. Such studies can reveal the PON-I enzyme's pharmacological potential to prevent atheroma formation [42].
Protective effects of PON-I enzyme against atherosclerosis
After infiltration to tunica media (stimulation by macrophage colony-stimulating factor and monocyte chemo attractant protein-1, monocytes absorb oxidized LDLs and eventually turn into foam cells. PON-I enzyme can inhibit the oxidation process of LDL by reactive oxygen species and thus reduces the number of oxidized lipoproteins. PON-I enzyme also plays a role in inhibiting the formation of atherosclerotic plaques with two mechanisms, first by increasing cholesterol excretion from macrophages and second by reducing monocyte chemo attractant protein-1 synthesis. With these two mechanisms, the PON-I enzyme can finally reduce the formation of foam cells [43].
PON-II
The PON-II enzyme is a high-expression intracellular protein with a molecular weight of 44 kDa [18]. Like the PON-I enzyme, the PON-II enzyme has several known polymorphisms involved in several pathophysiological conditions. Demographic surveys indicate replacing a pair of amino acids with alanine or glycine in codon 148 (a / g148) and cysteine or Serine in codon 311 (C / S311).
Studies have shown that A / G148 polymorphism is associated with changes in total cholesterol levels, fasting plasma glucose levels, and birth weight [44]. In addition, C/S311 polymorphism has been associated with CAD, ischemic shock in patients with type 2 diabetes, Alzheimer's, and decreased bone density in postmenopausal women [47, 48]. Although no information is available about the physiology and pathophysiology of the PON-II enzyme, it has been found that this protein has antioxidant potential. Studies have demonstrated that cells with high levels of the PON-II enzyme experience less oxidative stress when exposed to hydrogen peroxide and oxidized phospholipids [18].
Rasenblat et al. have also shown that purified PON-II can inhibit LDL oxidation [47]. In addition, the incubation of cells with high expression of PON-II enzyme and LDL showed less lipid peroxidation and lower induction of monocyte migration through endothelial cells. Thus, one of the PON-II enzyme's roles is acting as a cellular antioxidant and protecting cells against oxidative stress, although the exact mechanism is still unclear [18].
Regulation of PON-II enzyme expression
Unlike the PON-I enzyme, the level of PON-II expression and activity rises due to oxidative stress. Research has established that treating mice with oxidative stress can increase the expression of the PON-II enzyme and lactonase activity [47]. In humans, people with hypercholesterolemia have lower levels of the PON-II enzyme in monocyte-derived macrophages compared with people with normal cholesterol levels [48].
PON-III
PON-III enzyme is a 40 kDa protein accompanying HDL in the bloodstream, although it is lower than PON-I [49]. Between three members of the PON family, the PON-III enzyme is recently discovered, and there is not enough data available about it. Recently two polymorphisms have been found for PON-III in an Italian population. SNP (S / T311), in which threonine replaces serine in codon 311, and SNP (G / D324), in which aspartic acid replaces glycine in codon 324. The functional outcomes of these polymorphisms have not yet been determined [50]. PON-III enzyme has antioxidant potentials just like PON-I and PON-II enzymes. Studies by Dragano et al. have shown that purified PON-III enzyme can inhibit LDL oxidation Induced by copper [50]. It has also been discovered that the potential of the PON-III enzyme in inhibiting LDL oxidation can be 100 times more than PON-I. Unlike PON-I and PON-II enzymes which expression can change due to oxidative stress, the expression of the PON-III enzyme and its activity do not significantly change due to oxidative stress [16]. Since PON-III is the last enzyme discovered in the PON family, not much research has been done on the effect of its polymorphisms on atherosclerosis. However, for various reasons, such as low induction under oxidative stress, PON-III plays a lesser role in the risk of CAD than the other family members [51].
Conclusion
As mentioned, polymorphisms are different genotypes of a particular enzyme or protein that eventually produce different phenotypes with different characteristics. Due to the role of genetic factors in the development of atherosclerosis, many researchers decided to investigate the possible effect of polymorphisms of different genes on the risk of disease. Since oxidative stress plays a vital role in the development of atherosclerosis, research on oxidative stress defense systems became a turning point in these studies. Over the years, various polymorphisms of the PON enzyme, an essential component of the antioxidant defense system, have been identified. Studies have shown that some of these polymorphisms can strengthen or weaken the antioxidant defense system against oxidative stress by acting on enzymatic activity and therefore play a role in increasing or decreasing the risk of atherosclerosis.
In conclusion, research strongly shows that increasing the activity of PON enzymes, especially PON-I, will play an important role in reducing the risk of atherosclerosis by increasing resistance to oxidative stress [52]. Furthermore, there are different studies about the effect of polymorphisms on enzyme activity. Some of them revealed that specific polymorphisms with an effect on enzymatic activity ultimately increase or decrease the risk of disease in individuals, while in some populations, no association has been found between disease and polymorphism. Thus, further studies with a large population and meta-analyses in this field seem useful.
Conflict of Interests
The authors have no conflicts of interest to declare.
Acknowledgments
The authors would like to thank all involved in the study who encouraged us to do this project with their support. The authors would also like to thank the Shahid Sadoughi University of Medical Sciences for their support. This research received no external funding.
References
- Mack M, Gopal A. Epidemiology, traditional and novel risk factors in coronary artery disease. Heart failure Clinics 2016; 12(1): 1-10.
- Go AS, Mozaffarian D, Roger VL, Benjamin EJ, Berry JD, Blaha MJ, et al. Heart disease and stroke statistics 2014 update: a report from the American Heart Association. Circulation 2014; 129(3): 288-92.
- World Health Organization. Global health risks: mortality and burden of disease attributable to selected major risks: World Health Organization; 2009.
- Fava C, Montagnana M. Atherosclerosis is an inflammatory disease which lacks a common anti-inflammatory therapy: how human genetics can help to this issue. A narrative review. Frontiers in Pharmacol. 2018; 9(1): 55.
- Libby P, Okamoto Y, Rocha VZ, Folco E. Inflammation in atherosclerosis: transition from theory to practice. Circul J. 2010; 74(2): 213-20.
- Erbs S, Möbius-Winkler S, Linke A, Adams V, Doll N, Gielen S, et al. Both T-786C and G894T polymorphism of endothelial nitric oxide synthase affect in-vitro endothelium-dependent relaxation of internal mammary artery rings from patients with coronary artery disease. Euro J Prevent Cardiol. 2006; 13(5): 826-31.
- Ojima S, Kubozono T, Kawasoe S, Kawabata T, Miyata M, Miyahara H, et al. Association of risk factors for atherosclerosis, including high-sensitivity C-reactive protein, with carotid intima-media thickness, plaque score, and pulse wave velocity in a male population. Hyperten Res. 2020; 43(5): 422-30.
- Hirata A, Sugiyama D, Watanabe M, Tamakoshi A, Iso H, Kotani K, et al. Association of extremely high levels of high-density lipoprotein cholesterol with cardiovascular mortality in a pooled analysis of 9 cohort studies including 43,407 individuals: the EPOCH–JAPAN study. J Clinic Lipidol. 2018; 12(3): 674-84.
- Navab M, Ananthramaiah G, Reddy ST, Van Lenten BJ, Ansell BJ, Fonarow GC, et al. Thematic review series: the pathogenesis of atherosclerosis the oxidation hypothesis of atherogenesis: the role of oxidized phospholipids and HDL. J Lipid Res. 2004; 45(6): 993-1007.
- Nicholls SJ, Nelson AJ. HDL and cardiovascular disease. Pathology 2019; 51(2): 142-47.
- Lakshmy R, Ahmad D, Ann Abraham R, Kranthi Vemparala MS, Das S, Srinath Reddy K, et al. Paraoxonase gene Q192R & L55M polymorphisms in Indians with acute myocardial infarction & association with oxidized low density lipoprotein. India J Med Res. 2010; 131(4): 522.
- Shunmoogam N, Naidoo P, Chilton R. Paraoxonase (PON)-1: a brief overview on genetics, structure, polymorphisms and clinical relevance. Vascular Health and Risk Management 2018; 14(1): 137.
- Salari N, Rasoulpoor S, Hosseinian-Far A, Razazian N, Mansouri K, Mohammadi M, et al. Association between serum paraoxonase 1 activity and its polymorphisms with multiple sclerosis: a systematic review. Neurologic Sci. 2021; 42(2): 491-500.
- Nouryazdan N, Adibhesami G, Birjandi M, Heydari R, Yalameha B, Shahsavari G. Study of angiotensin-converting enzyme insertion/deletion polymorphism, enzyme activity and oxidized low density lipoprotein in Western Iranians with atherosclerosis: a case-control study. BMC Cardiovascular Disorders 2019; 19(1): 184.
- Draganov D, La Du BN. Pharmacogenetics of paraoxonases: a brief review. Naunyn-Schmiedeberg's Archiv Pharmacol. 2004; 369(1): 78-88.
- She ZG, Chen HZ, Yan Y, Li H, Liu DP. The human paraoxonase gene cluster as a target in the treatment of atherosclerosis. Antioxid Redox Signal. 2012; 16(6): 597-632.
- Reddy ST, Wadleigh DJ, Grijalva V, Ng C, Hama S, Gangopadhyay A, et al. Human paraoxonase-3 is an HDL-associated enzyme with biological activity similar to paraoxonase-1 protein but is not regulated by oxidized lipids. Arteriosclerosis, Thrombos Vascul Biol 2001; 21(4): 542-47.
- Ng CJ, Wadleigh DJ, Gangopadhyay A, Hama S, Grijalva VR, Navab M, et al. Paraoxonase-2 is a ubiquitously expressed protein with antioxidant properties and is capable of preventing cell-mediated oxidative modification of low density lipoprotein. J Biologic Chem. 2001; 276(48): 44444-4449.
- Zhou B, She J, Wang Y, Ma X. Venous thrombosis and arteriosclerosis obliterans of lower extremities in a very severe patient with 2019 novel coronavirus disease: a case report. J Thrombos Thrombolys. 2020; 50(1): 229-32.
- Demirdöğen BC, Akçin CK, Göksoy E, Yakar G, Öztepe T, Demirkaya-Budak S, et al. Paraoxonase 1 (PON1) promoter (-107T/C) and coding region (192Q/R and 55L/M) genetic variations in pseudoexfoliation syndrome and pseudoexfoliative glaucoma risk. Graefe's Arch Clinic Exper Ophthalmol. 2019; 257(10): 2257-270.
- Ng CJ, Shih DM, Hama SY, Villa N, Navab M, Reddy ST. The paraoxonase gene family and atherosclerosis. Free Radic Biol Med. 2005; 38(2): 153-63.
- Gupta N, Gill K, Singh S. Paraoxonases: structure, gene polymorphism & role in coronary artery disease. Indian Journal Of Medical Research 2009; 130(4): 215-22.
- Shahsavari G, Nouryazdan N, Adibhesami G, Birjandi M. Genetic associations and serum paraoxonase levels with atherosclerosis in western Iranian patients. Mol Biol Rep. 2020; 47(7): 5137-144.
- Amini M, Esmaeilzadeh-Bahabadi S, Avan A, Gholoobi A, Ghasemi F, Mirhafez SR, et al. Paraoxonase-1 Q192R polymorphism and its association with hs-CRP and fasting blood glucose levels and risk of coronary artery disease. Diabetes Metabolic Syndrome: Clinic Res Rev. 2019; 13(2): 1053-1057.
- Saadat M. Evaluation of association between Q192R and L55M genetic polymorphisms of PON1 and serum paraoxonase-1 activity in healthy individuals, a meta-analysis. Romanian Journal of Diabetes Nutr Metabol Dis. 2018; 25(2): 171-80.
- Mackness MI, Mackness B, Durrington PN. Paraoxonase and coronary heart disease. Atherosclerosis Supplements 2002; 3(4): 49-55.
- Sentı M, Tomas M, Anglada R, Elosua R, Marrugat J, Covas MI, et al. Interrelationship of smoking, paraoxonase activity, and leisure time physical activity: a population-based study. Euro J Int Med. 2003; 14(3): 178-84.
- Malakar AK, Choudhury D, Halder B, Paul P, Uddin A, Chakraborty S. A review on coronary artery disease, its risk factors, and therapeutics. J Cell Physiol. 2019; 234(10): 16812-6823.
- Jarvik GP, Tsai NT, McKinstry LA, Wani R, Brophy VH, Richter RJ, et al. Vitamin C and E intake is associated with increased paraoxonase activity. Arterioscl, Thromb, Vascul Biol. 2002; 22(8): 1329-333.
- Deakin S, Leviev I, Guernier S, James RW. Simvastatin modulates expression of the PON1 gene and increases serum paraoxonase: a role for sterol regulatory element–binding protein-2. Arterioscl, Thromb, Vascul Biol. 2003; 23(11): 2083-2089.
- Sierksma A, van der Gaag MS, van Tol A, James RW, Hendriks HF. Kinetics of HDL cholesterol and paraoxonase activity in moderate alcohol consumers. Alcoholism: Clinic Experiment Res. 2002; 26(9): 1430-435.
- Marsillach J, Camps J, Ferré N, Beltran R, Rull A, Mackness B, et al. Paraoxonase-1 is related to inflammation, fibrosis and PPAR delta in experimental liver disease. BMC Gastroenterol. 2009; 9(1): 1-13.
- Mahrooz A, Mackness M, Bagheri A, Ghaffari-Cherati M, Masoumi P. The epigenetic regulation of paraoxonase 1 (PON1) as an important enzyme in HDL function: The missing link between environmental and genetic regulation. Clinic Biochem. 2019;73(1): 1-10.
- Ferretti G, Bacchetti T. Effect of dietary lipids on paraoxonase-1 activity and gene expression. Nutrition, Metabol Cardiovascul Dis. 2012; 22(2): 88-94.
- Kupczyk D, Bilski R, Sokołowski K, Pawłowska M, Woźniak A, Szewczyk-Golec K. Paraoxonase 1: the lectin-like oxidized LDL receptor type I and oxidative stress in the blood of men with type II obesity. Dis Marker 2019; 6178017: 1-7.
- Aviram M, Billecke S, Sorenson R, Bisgaier C, Newton R, Rosenblat M, et al. Paraoxonase active site required for protection against LDL oxidation involves its free sulfhydryl group and is different from that required for its arylesterase/ paraoxonase activities: selective action of human paraoxonase allozymes Q and R. Arteriosclerosis, Thrombosis, and Vascular Biology. 1998; 18(10): 1617-624.
- Harel M, Aharoni A, Gaidukov L, Brumshtein B, Khersonsky O, Meged R, et al. Structure and evolution of the serum paraoxonase family of detoxifying and anti-atherosclerotic enzymes. Nat Struct Mol Biol. 2004; 11(5): 412-19.
- Ayan D, Şeneş M, Çaycı AB, Söylemez S, Eren N, Altuntaş Y, et al. Evaluation of paraoxonase, arylesterase, and homocysteine thiolactonase activities in patients with diabetes and incipient diabetes nephropathy. J Med Biochem. 2019; 38(4): 481-89.
- Mackness MI, Arrol S, Abbott C, Durrington PN. Protection of low-density lipoprotein against oxidative modification by high-density lipoprotein associated paraoxonase. Atherosclerosis 1993; 104(1-2): 129-35.
- Brites F, Martin M, Guillas I, Kontush A. Antioxidative activity of high-density lipoprotein (HDL): Mechanistic insights into potential clinical benefit. BBA Clinical. 2017; 8(1): 66-77.
- Doorn JA, Sorenson RC, Billecke SS, Hsu C, La Du BN. Evidence that several conserved histidine residues are required for hydrolytic activity of human paraoxonase/ arylesterase. Chemico-Biological Interactions 1999; 119: 235-41.
- Oda MN, Bielicki JK, Ho TT, Berger T, Rubin EM, Forte TM. Paraoxonase 1 overexpression in mice and its effect on high-density lipoproteins. Biochem Biophysic Res Communic. 2002; 290(3): 921-27.
- Kulka M. A review of paraoxonase 1 properties and diagnostic applications. Polish Journal Of Veterinary Sciences. 2016; 19(1): 225-32.
- Qujeq D, Mahrooz A, Alizadeh A, Boorank R. Paraoxonase-2 variants potentially influence insulin resistance, beta-cell function, and their interrelationships with alanine aminotransferase in type 2 diabetes. J Res Med Sci. 2018; 23(12): 107.
- Chen Q, Reis SE, Kammerer CM, McNamara DM, Holubkov R, Sharaf BL, et al. Association between the severity of angiographic coronary artery disease and paraoxonase gene polymorphisms in the National Heart, Lung, and Blood Institute–sponsored Women’s Ischemia Syndrome Evaluation (WISE) Study. Am J Human Genet. 2003; 72(1): 13-22.
- Janka Z, Juhasz A, Rimanoczy A, Boda K, Marki-Zay J, Kalman J. Codon 311 (Cys→ Ser) polymorphism of paraoxonase-2 gene is associated with apolipoprotein E4 allele in both Alzheimer's and vascular dementias. Mol Psychiatry. 2002; 7(1): 110-12.
- Rosenblat M, Draganov D, Watson CE, Bisgaier CL, La Du BN, Aviram M. Mouse macrophage paraoxonase 2 activity is increased whereas cellular paraoxonase 3 activity is decreased under oxidative stress. Arteriosclerosis, Thromb, Vascular Biol. 2003; 23(3): 468-74.
- Rosenblat M, Hayek T, Hussein K, Aviram M. Decreased macrophage paraoxonase 2 expression in patients with hypercholesterolemia is the result of their increased cellular cholesterol content: effect of atorvastatin therapy. Arterioscl, Thromb, Vascul Biol. 2004; 24(1): 175-80.
- Draganov DI, Stetson PL, Watson CE, Billecke SS, La Du BN. Rabbit serum paraoxonase 3 (PON3) is a high density lipoprotein-associated lactonase and protects low density lipoprotein against oxidation. J Biol Chem. 2000; 275(43): 33435-34342.
- Campo S, Sardo AM, Campo GM, Avenoso A, Castaldo M, D’Ascola A, et al. Identification of paraoxonase 3 gene (PON3) missense mutations in a population of southern Italy. Mutation Research/Fundamental Molecular Mechanisms Mutagenesis 2004; 546(1-2): 75-80.
- Ng CJ, Shih DM, Hama SY, Villa N, Navab M, Reddy ST. The paraoxonase gene family and atherosclerosis. Free Radical Biol Med. 2005; 38(2): 153-63.
- Chistiakov DA, Melnichenko AA, Orekhov AN, Bobryshev YV. Paraoxonase and atherosclerosis-related cardiovascular diseases. Biochimie 2017; 132(1): 19-27.