Department of Microbiology, Faculty of Medicine, Iran University of Medical Sciences, Tehran, Iran
Full-Text [PDF 608 kb]
(362 Downloads)
|
Abstract (HTML) (933 Views)
Full-Text: (344 Views)
Microbiological culture is a primary method of letting organisms multiply in culture media under controlled in vitro conditions [1]. This technique is used to determine the type of organism in specimens isolated from patients such as blood, wound discharge, bronchial, pleural or ascitic fluid, etc., in order to determine the probable cause of infection [2].
Antimicrobial resistance is not new; however, the magnitude of resistant organisms, the geographic locations affected by drug resistance, and the breadth of resistance in single organisms are unprecedented and mounting [3]. Over time and due to a wide variety of causes, different bacteria can acquire resistance to various antibiotics [4]. An important task of clinical microbiology laboratory is the antimicrobial susceptibility testing of bacterial isolates from clinical specimens [5]. The tests will detect possible drug resistance in common pathogens and ensure susceptibility to drugs of choice for particular infections. They are performed to determine which antibiotic will most successfully treat a bacterial infection in vivo as susceptibility can vary even within a species [6, 7]. Diseases once thought to be controlled by particular antibiotics are becoming increasingly resistant to standard therapies. Drug-resistant strains initially appeared in hospitals where most antibiotics were being used [8]. Resistance to multiple drugs was first detected among enteric bacteria, namely, E. coli, Shigella spp., and Salmonella spp. in the late 1950s to early 1960 [9, 10]. The use of broad-spectrum antibiotics has been the most important cause of antibiotic resistance in recent years [11]. The emergence of resistant bacteria and the problems these persistent bacteria cause in treating patients urge the necessity for precise knowledge about these bacteria and their susceptibility patterns [4, 11, 12]. Attention to microbial antibiotic responses can help control disease outbreak in hospital settings [13].
Administration of effective antibiotics not only can improve the quality of patient treatment but also can reduce medical costs. This study aimed to determine the antibiotic susceptibility patterns of bacterial isolates from patients in three different hospitals in Yazd city, Iran.
Materials and Methods
Study Area
Clean catch clinical specimens were obtained from 336 patients (223 men and 113 women) referred to different wards of three hospitals in Yazd city, Iran. The study was conducted during ten months period (from March 2015 to January 2016). All experiments were performed in the department of microbiology laboratory, faculty of medicine, Shahid Sadoughi University of Medical Sciences, Yazd, Iran.
Sample collection / Study design
Patient specimens which included wound discharge, sputum, blood, bronchial fluid, pleural fluid, ascitic fluid, synovial fluid, pleural fluid, stool, and trachea secretions were collected from 336 hospitalized patients. Selections were made irrespective of the infection's cause, and patient age ranged from 6 to 87 years. The exclusion criteria were the lack of antibiotic therapy prior to sample collection.
Each sample was collected in a 20 ml calibrated sterile container. The specimens were appropriately labeled, transported to the laboratory immediately, and analyzed within 3 hours after collection.
Sample processing/culture and identification of organisms
Culture and antibiotic susceptibility tests were performed on clinical specimens obtained from the patients before initiation of any antibiotic therapy. Each sample was aseptically inoculated into blood agar and MacConkey agar differential media on arrival at the laboratory. The plates were incubated aerobically at 37 °C for 24-48 hours. Then, the characteristic bacterial isolates observed on the differential media plates were sub-cultured onto new culture media plates. Any grown bacteria were subjected to microscopical and appropriate selective tests such as catalase and coagulase for Gram-positive and IMVIC for gram-negative bacteria to identify each organism correctly.
Antibiotic susceptibility test
Bacterial identification was made based on cultural and biochemical characteristics [14]. The following antibiotic disks were used for each bacterium based on their Gram stain and clinical sources: Tetracycline (30μg), Ceftriaxone (30μg), Ciprofloxacin (5μg), Ceftizoxime (30μg), Amikacin (30μg), Chloramphenicol (30μg), Cephalothin (30μg), Nalidixic acid (30μg), Ceftazidime (30μg), Amoxicillin-clavlanate (30μg), Imipenem (10μg), Ampicillin (10μg), Norfloxacin (10μg), Nitrofurantoin (300μg), Carbenicillin (100μg), Gentamicin (10μg), Meropenem (10μg), Clarithromycin (15μg), Clindamycin (2μg), Azithromycin (15μg), Vancomycin (30μg), Oxacillin (1μg), Penicillin (10μg), Polymyxin B (300μg), Piperacillin (100μg), Doxycycline (30μg), Ticarcillin (75μg), Cefotaxime (30μg), Cefazolin (30μg), Cefuroxime (30μg), Cefepime (30μg), Cefixime (5μg), Ofloxacin (5μg), Tobramycin (10μg), Streptomycin (10μg), Cefoxitin (30μg), Colistin (10μg), Rifampin (5μg), Trimethoprim-Sulfamethoxazole (1.25/23.75μg), Levofloxacin (5μg), Fosfomycin (200μg), Ampicillin-Sulbactam (10/10μg), Aztreonam (30μg), Trimethoprime (5μg), and Erythromycin (15μg). In the disk diffusion assay used in this study, within 15 min after applying the antibiotic discs (Oxoid Co., Australia), the plates were incubated at 37 °C. After 24h of incubation, plates were examined, and the diameter of growth inhibition zones was measured. The zone diameter for individual antimicrobial agents was then translated into susceptible, intermediate, and resistant categories based on Clinical and Laboratory Standards Institute 2015 criteria.
Institutional Review Board approved this study, so there was no deviation from the prescribed standard protocol of treatment, and it was conducted after obtaining due ethical approval from hospital administration.
Results
The present study includes culture information and antimicrobial susceptibility data for each bacterial isolate obtained from patients hospitalized for 72 hrs or more in hospitals of Yazd. The age of patients in this study ranged from newborn to 87 years (Figure 1).
The distribution of patients from whom specimens were taken in each hospital ward is shown in Table 1. From the 336 positive urine cultures, 22 different bacterial isolates were obtained. E. coli was the predominant and most frequently isolated pathogen, followed by S. aureus. Figure 2 shows the details of cultured bacteria. Among all the organisms isolated from patients, the eight most frequent organisms were chosen and subjected to an antibiotic susceptibility test. Bacterial susceptibility to tetracycline and Ceftriaxone was summarized in Table 2, and susceptibility to ampicillin and gentamicin was shown in Table 3.
Table 1. Distribution and number of patients in different hospital wards
Part of hospital |
Number of patients |
Intensive care unit |
73 |
Internal |
49 |
Surgery |
66 |
Cardiac care unit |
4 |
Very important person |
7 |
Gynecology |
2 |
New born |
6 |
Pediatrics |
2 |
Neonatal intensive care unit |
4 |
Infectious |
86 |
Urology |
14 |
Surgical intensive care unit |
10 |
Central lab |
13 |
Total |
336 |
Table 2. Susceptibility to Tetracycline and Ceftriaxone
|
Tetracyclin |
Ceftriaxone |
Pathogen |
Intermediate |
Sensitive |
Resistant |
Intermediate |
Sensitive |
Resistant |
E.coli |
2 |
33 |
25 |
9 |
21 |
30 |
Staph aureus |
3 |
18 |
33 |
24 |
9 |
21 |
Acinetobacter.sp |
2 |
19 |
24 |
3 |
5 |
37 |
Pseudomonas |
20 |
0 |
8 |
10 |
6 |
12 |
Staph epidermidis |
7 |
11 |
6 |
3 |
17 |
4 |
Staph suprophyticus |
8 |
25 |
14 |
12 |
19 |
16 |
Klebsiella.sp |
6 |
5 |
15 |
5 |
8 |
13 |
Enterococci.sp |
5 |
4 |
4 |
1 |
7 |
5 |
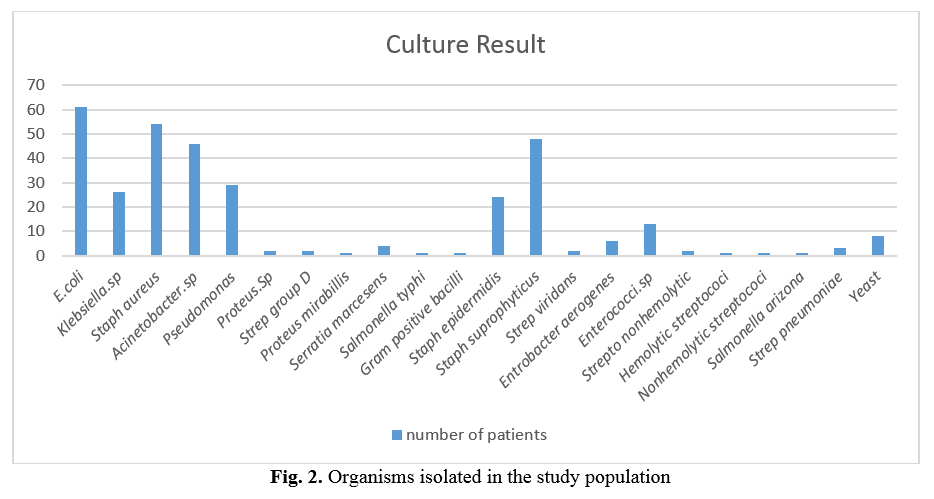
Table 3. Susceptibility to Ampicillin and Gentamicin
|
Ampicilin |
Gentamicin |
Pathogen |
Intermediate |
Sensitive |
Resistant |
Intermediate |
Sensitive |
Resistant |
E.coli |
9 |
10 |
41 |
12 |
28 |
20 |
Staph aureus |
6 |
14 |
37 |
16 |
33 |
8 |
Acinetobacter.sp |
5 |
3 |
37 |
3 |
3 |
39 |
Pseudomonas |
5 |
3 |
21 |
5 |
20 |
4 |
Staph epidermidis |
4 |
7 |
13 |
0 |
19 |
5 |
Staph suprophyticus |
8 |
14 |
25 |
9 |
21 |
17 |
Klebsiella.sp |
6 |
2 |
18 |
1 |
17 |
8 |
Enterococci.sp |
3 |
6 |
4 |
0 |
8 |
4 |
Discussion
Infectious diseases by resistant bacteria have become an extraordinary predicament in all medical and therapeutic centers [15]. The appearance of antibiotic-resistant bacterial strains has become a worldwide danger [16, 17]. Development of antibiotic resistance in particular stems from drugs targeting only specific bacterial molecules [18]. Because the drug is so specific, any mutation in these molecules will interfere with or negate its destructive effect, resulting in antibiotic resistance [19, 20]. Many investigators have demonstrated that E. coli, S. aureus, Pseudomonas spp., and Klebsiella spp. are frequently isolated from hospitalized patients [21-24]. In the present study, eight organisms were more common among the 23 organisms isolated from patients. Sixty patients from 336 showed evidence of urinary tract infections (UTI) due to E. coli (17.8%). S. aureus was the next prevalent isolate (16.9%), followed by S. saprophyticus (13.9%), Acinetobacter spp. (13.3%), Pseudomonas spp. (8.6%), Klebsiella spp. (7.7%), S. epidermidis (7.1%) and Enterococcus spp. (3.8%), respectively. Most patients in our study were between 71 to 80 years of age (21.7%). Furthermore, they were mostly hospitalized in infectious diseases wards (25.5%).
When whole populations are treated with the same class of antibiotics, susceptible strains will have little opportunity to recolonize their niche, and resistant strains will acquire an important advantage [25, 26]. E. coli is an increasingly prevalent antibiotic-resistant Gram-negative bacterium in hospitals. This bacterium causes UTI, gastroenteritis, septicemia, and meningitis. The E. coli isolates in this study were uniformly resistant to beta-lactam antibiotics such as ampicillin (68% of all isolated E. coli) and Ceftriaxone (50% of all isolated E. coli; however, they were somewhat sensitive to tetracycline and gentamicin. The S. aureus isolates were sensitive to gentamicin (70.2% of all isolated S. aureus), very resistant to tetracycline (61.1%), and have shown outstanding intermediate resistance to Ceftriaxone (44.4%). Acinetobacter isolates were resistant to almost all the antibacterial agents. S. saprophyticus isolates were sensitive to tetracycline (53.2%), ceftriaxone (40.4%), and gentamicin (44.6%) but resistant to ampicillin (53.1%). Pseudomonas isolates were sensitive to gentamicin (68.9%) while resistant to ampicillin, ceftriaxone, and tetracycline. The same organism in different wards of hospitals shows different antibiotic resistance patterns. This may be due to three main reasons:
first, drug inactivation or modification
(e.g., enzymatic deactivation of penicillin G in some penicillin-resistant bacteria through the production of β-lactamases). Second, alteration of the target site (e.g., alteration of the binding target site for penicillin). Third, alteration of metabolic pathways [26-28].
Conclusion
The increased prevalence of resistant organisms in different hospitals may reflect a lack of proper antibiotic usage policy, resulting in the prolonged and indiscriminate use of antimicrobial agents.
Conflict of interest
The authors declare have no conflict of interest to declare.
Acknowledgements
Not applicable.
References
- Donaldson A, McKenzie D, Riggio M, Hodge P, Rolph H, Flanagan A, et al. Microbiological culture analysis of the tongue anaerobic microflora in subjects with and without halitosis. Oral Diseases 2005; 11(S1): 61-3.
- Benson HJ. Microbiological applications: laboratory manual in general microbiology: McGraw-Hill Science, Engineering & Mathematics; 2002.
- Levy SB, Marshall B. Antibacterial resistance worldwide: causes, challenges and responses. Nature Medicine 2004;10(12 Suppl): S122-29.
- Levy SB. The challenge of antibiotic resistance. Scientific American 1998; 278(3): 32-9.
- Reller LB, Weinstein M, Jorgensen JH, Ferraro MJ. Antimicrobial susceptibility testing: a review of general principles and contemporary practices. Clinical Infectious Diseases 2009; 49(11): 1749-455.
- Barenfanger J, Drake C, Kacich G. Clinical and financial benefits of rapid bacterial identification and antimicrobial susceptibility testing. J Clinic Microbiol. 1999; 37(5): 1415-418.
- Karlowsky JA, Jones ME, Draghi DC, Thornsberry C, Sahm DF, Volturo GA. Prevalence and antimicrobial susceptibilities of bacteria isolated from blood cultures of hospitalized patients in the United States in 2002. Ann Clinic Microbiol. Antimicrob. 2004; 3(1): 7.
- Dominey-Howes D, Labbate M. The antimicrobial resistance crisis: causes, consequences, and management. Front Public Health 2014; 2: 145.
- Landecker H. Antibiotic resistance and the biology of history. Body Society 2016; 22(4): 19-52.
- Canizalez-Roman A, Gonzalez-Nuñez E, Vidal JE, Flores-Villaseñor H, León-Sicairos N. Prevalence and antibiotic resistance profiles of diarrheagenic Escherichia coli strains isolated from food items in northwestern Mexico. Int J Food Microbiol. 2013; 164(1): 36-45.
- Van Boeckel TP, Gandra S, Ashok A, Caudron Q, Grenfell BT, Levin SA, et al. Global antibiotic consumption 2000 to 2010: an analysis of national pharmaceutical sales data. Lancet Infect Dis. 2014; 14(8): 742-50.
- Potron A, Poirel L, Nordmann P. Emerging broad-spectrum resistance in Pseudomonas aeruginosa and Acinetobacter baumannii: mechanisms and epidemiology. Int J Antimicrob Agents. 2015; 45(6): 568-85.
- Edelsberg J, Weycker D, Barron R, Li X, Wu H, Oster G, et al. Prevalence of antibiotic resistance in US hospitals. Diagnostic Microbiol Infect Dis. 2014; 78(3): 255-62.
- Jorgensen JH, Turnidge JD. Susceptibility test methods: dilution and disk diffusion methods. Manual of Clinical Microbiology, 11th ed. American Society of Microbiology, 2015; p. 1253-273.
- Jones KE, Patel NG, Levy MA, Storeygard A, Balk D, Gittleman JL, et al. Global trends in emerging infectious diseases. Nature 2008; 451(7181): 990-93.
- Xilin Z, Drlica K. Restricting the selection of antibiotic-resistant mutant bacteria: measurement and potential use of the mutant selection window. J Infect Dis. 2002; 185(4): 561-65.
- Khachatourians GG. Agricultural use of antibiotics and the evolution and transfer of antibiotic-resistant bacteria. Canad Med Assoc J. 1998; 159(9): 1129-136.
- Mansour SC, Hancock RE. Peptide IDR‐1018: modulating the immune system and targeting bacterial biofilms to treat antibiotic‐resistant bacterial infections. J Peptid Sci. 2015; 21(5): 323-29.
- Rizzo L, Manaia C, Merlin C, Schwartz T, Dagot C, Ploy M, et al. Urban wastewater treatment plants as hotspots for antibiotic resistant bacteria and genes spread into the environment: a review. Sci Total Environ. 2013; 447: 345-60.
- Sharma VK, Johnson N, Cizmas L, McDonald TJ, Kim H. A review of the influence of treatment strategies on antibiotic resistant bacteria and antibiotic resistance genes. Chemosphere 2016; 150: 702-14.
- Sader HS, Rhomberg PR, Farrell DJ, Jones RN. Arbekacin activity against contemporary clinical bacteria isolated from patients hospitalized with pneumonia. Antimicrob Agent Chemother. 2015; 59(6): 3263-270.
- Sánchez CJ, Mende K, Beckius ML, Akers KS, Romano DR, Wenke JC, et al. Biofilm formation by clinical isolates and the implications in chronic infections. BMC Infect Dis. 2013; 13(1): 47.
- Sader HS, Farrell DJ, Flamm RK, Jones RN. Antimicrobial susceptibility of Gram-negative organisms isolated from patients hospitalised with pneumonia in US and European hospitals: results from the SENTRY Antimicrobial Surveillance Program, 2009-2012. Int J Antimicrob Agents 2014; 43(4): 328-34.
- Warnke PH, Lott AJ, Sherry E, Wiltfang J, Podschun R. The ongoing battle against multi-resistant strains: in-vitro inhibition of hospital-acquired MRSA, VRE, Pseudomonas, ESBL E. coli and Klebsiella species in the presence of plant-derived antiseptic oils. J Cranio-Maxillofac Surg. 2013; 41(4): 321-26.
- Borges A, Ferreira C, Saavedra MJ, Simoes M. Antibacterial activity and mode of action of ferulic and gallic acids against pathogenic bacteria. Microb Drug Resist. 2013; 19(4): 256-65.
- Latif S, Ahmad I. Bacterial pathogens responsible for blood stream infection (BSI) and pattern of drug resistance in a tertiary care hospital of Lahore. Biomedica 2017; 25(2): 101-105.
- Toprak E, Veres A, Yildiz S, Pedraza JM, Chait R, Paulsson J, et al. Building a morbidostat: an automated continuous-culture device for studying bacterial drug resistance under dynamically sustained drug inhibition. Nat Protocols 2013; 8(3): 555-67.
- Ashton PM, Nair S, Dallman T, Rubino S, Rabsch W, Mwaigwisya S, et al. MinION nanopore sequencing identifies the position and structure of a bacterial antibiotic resistance island. Nat Biotechnol. 2015; 33(3): 296-300.
Type of Study:
Research |
Subject:
Bactriology Received: 2022/03/16 | Accepted: 2021/03/29 | Published: 2021/03/29